Contents:
Synapse structure and function
CHEMICAL SYNAPSES
- Most signaling between nerve cells occurs via chemical synapses.
- These are formed by the close association of an axon terminal of the presynaptic cell with some part of the postsynaptic cell.
- The gap between the cells, the synaptic cleft, is typically 20 nm across though in some synapses can be much wider.
- Synapses are classified by where on the receiving cell they are located. Most are axodendritic synapses made on dendrites; on spiny dendrites, synapses are formed on spines.
- Particularly powerful are axosomatic synapses made on the cell body (named for an alternative term for the nerve cell body, the soma).
- Synapses between axon terminals and axons of postsynaptic neurons are axoaxonal synapses.
MORPHOLOGY OF CHEMICAL SYNAPSES
- Electron microscopy has revealed numerous morphologically distinct types of synapses, but all share common features.
- Spherical, clear, small synaptic vesicles (SSVs), 50 nm across, store neurotransmitters and are scattered throughout the terminal in association with microtubules that transport them to the presynaptic membrane.
- The presynaptic membrane is thickened and may have dense projections which are involved in the docking of synaptic vesicles at the active zone, the region from which transmitter release occurs.
- The dendrite cell membrane at the synapse is thickened as the postsynaptic density, formed by the proteins that constitute the postsynaptic machinery.
- Cortical axodendritic synapses are asymmetrical in that they have an extremely well-developed postsynaptic density, they are usually excitatory.
- Axosomatic synapses are symmetrical in having pre-and postsynaptic densities of comparable thickness, they are usually inhibitory. The cerebral cortex may have as many as 1013 synapses.
- Some synapses lack obviously specialized contact zones on both pre-and postsynaptic sides and have extremely wide (100–500 nm) synaptic clefts.
- These often secrete a catecholamine and have large dense-core vesicles (LDCVs) 40–120 nm across. LDCVs are also found in peptide-secreting neurons.
- Many synapses contain both SSVs and LDCVs. This structural evidence supports physiological studies showing that many neurons secrete more than one transmitter.
CHEMICAL TRANSMISSION
- Most synapses are chemical. At a typical CNS synapse, the arrival of an action potential at the axon terminal may, or may not, trigger the release of neurotransmitters from a single presynaptic vesicle.
- Transmitter release requires a rise in intracellular Ca2+ brought about by calcium entry into the axon terminal via voltage-dependent calcium channels.
- The transmitter diffuses across the synaptic cleft in about 5 μs. At the postsynaptic membrane, it binds to specific receptors, causing a change in the receptor conformation.
- What happens next depends on the receptor, but the overall result is to change the postsynaptic membrane permeability to specific ions.
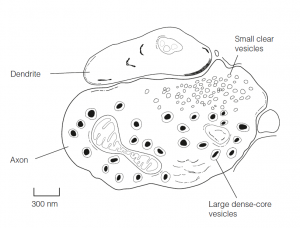
- Neurotransmitter receptors come in two superfamilies.
- The ligand-gated ion channels (LGICs), also referred to as ionotropic receptors, have ion-selective channels as part of the receptor.
- The binding of the transmitter to the receptor opens the channel, directly increasing its permeability.
- The second superfamily is the G-protein-coupled receptors (GPCRs). These are the largest group of metabotropic receptors, so-called because they can modulate metabolic activities.
- The binding of the transmitter to these receptors activates their associated G proteins that are capable of diverse and remote effects on membrane permeability, excitability, and metabolism.
- G proteins can influence permeability either by binding ion channels directly or by modifying the activity of second messenger system enzymes which phosphorylate ion channels, thereby altering their permeability.
- The changes in membrane properties brought about by a transmitter produce postsynaptic potentials, small shifts in the membrane potential that can have essentially one of two effects.
- It may increase the probability that the postsynaptic neuron fires action potentials, in which case the response is excitatory.
- If the effect is to decrease the probability that the postsynaptic cell might fire, the response is inhibitory.
- It is commonly the case that a transmitter is excitatory at one of its receptors, but inhibitory at another, so it is a synapse that is excitatory or inhibitory rather than the transmitter per se.
- Numerous molecules have been identified as neurotransmitters.
- The classical transmitters are small molecules, amino acids, or amines. Quantitatively by far, the most important is glutamate, which is almost invariably excitatory, and g-aminobutyrate (GABA) which is usually inhibitory. This group also includes acetylcholine, the catecholamines such as dopamine and noradrenaline (norepinephrine), and the indoleamine, serotonin. A second, larger, group is an eclectic mix of peptides which includes the opioids (such as endorphins) and tachykinins (e.g., substance P).
- Fast transmission occurs whenever a neurotransmitter acts via LGICs, whereas slow transmission occurs when transmitters act at GPCRs.
- Glutamate, GABA, and acetylcholine (ACh) are together responsible for most fast transmission.
- However, each of these molecules also mediates slow transmission by activating their corresponding GPCR. Indeed, a transmitter can mediate both fast and slow transmission at the same synapse by activating multiple receptor populations.
- For example, ACh released from preganglionic cells acts on both nicotinic and muscarinic receptors on postganglionic cells in autonomic ganglia, mediating fast and slow effects of ACh respectively.
- Catecholamine and peptide transmission are invariably slow.
- It is extremely common for a given synapse to release more than one transmitter.
- This transmission usually involves the release of a classical transmitter, coupled with the co-release of one or more peptides at higher firing frequencies.
- Transmitters are rapidly cleared from the synaptic cleft after release by passive diffusion away from the cleft, reuptake into surrounding neurons or glia, or enzyme degradation.
ELECTRICAL TRANSMISSION
- Electrical transmission is mediated by electrical synapses, which are gap junctions between adjacent neurons. Gap junctions are arrays of paired hexameric ion channels called connexons.
- The channel pores are 2–3 nm in diameter, allowing ions and small molecules to permeate between neighboring neurons.
- By electrically coupling neurons, gap junctions allow any potentials, for example, action potentials, to spread between cells.
- Key features of electrical transmission are that it is extremely rapid, signals are transmitted with no distortion, and it works in both directions.
- Gap junctions between cells can close. Each connexon is made up of six subunits called connexins, and in response to a rise in intracellular Ca2+ concentration, the connexins rotate to close the central pore.
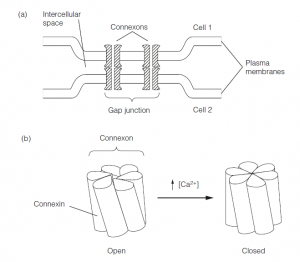
References
- https://epdf.pub/bios-instant-notes-in-neurosciencec1fc0c470dd0ec4352742d82b187650069114.html
- https://books.google.com/books?id=IvJ4AgAAQBAJ